The following is an excerpt (Chapter 3) from an Issue Paper published by the Council for Agricultural Science and Technology: Food Biofortification — Reaping the Benefits of Science to Overcome Hidden Hunger, No. 69, Oct. 2020.
The initial question facing plant breeders was: Could high iron, zinc, and vitamin A density be combined with high yields and profits?
While traditional crop improvement focuses on value added traits for existing markets that provide superior crop and/or marketing options to farmers, biofortification breeding adds traits which positively affect human health to these product profiles. This entails integration of nutrition in setting target levels or defining standards based on the likely contribution of these traits to nutritional status in defining trait values. In parallel, coordinated marketing research needs to be conducted in order to assure that requirements and value propositions for all value chain actors are incorporated in product profiles. Once early proof-of-concept research confirmed the feasibility that breeding can add the required nutrient target levels to staple crops, breeding for micronutrient density assumed full operational scale under HarvestPlus during 2007–2010. Initial and progressive waves of biofortified varieties now are planted by more than 10 million farmers in more than 30 countries.
The crop development process entails screening germplasm for available genetic diversity, pre-breeding parental genotypes, developing and testing micronutrient-dense germplasm, conducting genetic studies, applying rapid generation advance through “speed breeding” and developing and applying molecular markers and genomic selection strategies to lower costs, accelerate pace, and boost rates of genetic gain. Once promising lines or hybrids are developed, they are tested in numerous locations across target environments to assess genotype x environment interaction (GxE)—the influence of the growing environment on micronutrient levels, agronomic performance and end-use traits of the varieties or hybrids tested. Large scale regional GxE testing now enables reduced time-to-market for biofortified varieties by using spatial environmental variation to substitute for temporal variation, and eliminating testing steps.
Setting Nutrient Targets
Early in the conceptual development of biofortification, a working group of nutritionists, food technologists, and plant breeders established nutritional breeding targets by crop, based on food consumption patterns of target populations, estimated nutrient losses during storage and processing, and nutrient bioavailability (Hotz and McClafferty 2007). As with commercial fortification, breeding targets for biofortified crops were designed to meet biologically important proportions of the specific dietary needs of women and children. The answer is complex, depending on age- and gender-specific nutrient requirements, per capita consumption of a particular food, bioavailability of the nutrients, and nutrient retention—as shown in the equation on page 18.
Table 7 below shows the target increments for plant breeding and the incremental percentages of the EAR provided by biofortified crops for preschool children 4–6 years old and for non-pregnant, non-lactating women of reproductive age.
In addition to direct breeding for higher nutrient levels, genotypic differences in bioavailability and retention offer potential to contribute to effectively achieving targets through indirect breeding if genetic variation and prerequisite criteria to address these relevant complex traits through breeding exist. Breeding for micronutrient bioavailability per se is greatly limited by the lack of large-scale, rapid in vitro and/or animal bioavailability models for germplasm evaluation. Hence, dissecting overall bioavailability into its causal components and selectable traits, such as anti-nutrients and promoters, is a common current approach.
Measuring Micronutrient Densities
Existing genetic variation, trait heritability, gene action, associations among traits, available screening techniques, and diagnostic tools are commonly considered and used to identify selectable traits and estimate potential genetic gains through breeding. Biofortification breeding required developing or adapting cost-effective and rapid high throughput analytical techniques for micronutrients, as thousands of samples need to be tested for mineral or vitamin content each season. In parallel with screening, inexpensive analytical methods for high throughput micronutrient screening were tested and further developed along with non-contaminating milling and grinding equipment to boost breeding effectiveness (Yasmin et al. 2014). Contamination in mineral analyses—for example, by iron—resulting from soil, dust, or threshing equipment can be detected using indicator elements aluminum, titanium, and chromium that that are abundant in nature, but absent or present only in trace amounts in plants or seed. Factors that pose challenges to sampling and trait diagnostics are short sample analysis turnaround requirements for crops with two or more cycles per year and rapid post-harvest deterioration, particularly tuber crops or fruits, which are harvested with high moisture content. In contrast to minerals which are very stable, provitamin A carotenoids undergo significant degradation during storage, drying, milling, and processing.
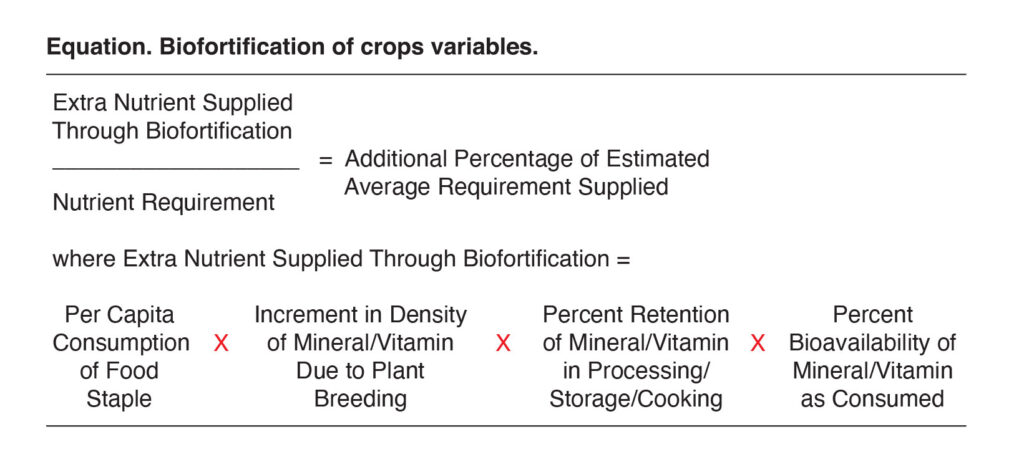
Next to precision analysis methods for minerals (such as Inductively Coupled Plasma Argon Optical Emission Spectrometer; ICP), the high throughput X-Ray Fluorescence Spectrometer (XRF) method has been adapted to analysis of plant samples (Guild et al. 2017; Guild and Stangoulis 2016; Paltridge et al. 2012a; Paltridge et al. 2012b; Sosa et al. 2018). XRF requires minimal pre-analysis preparation and allows for non-destructive analysis and was widely implemented. Due to its sensitivity and selectivity, high performance liquid chromatography (HPLC) is the method of choice to quantify individual carotenoids and their isomers in grains, whereas Near Infrared Reflectance Spectrophotometry (NIRS) is widely used for root and tuber crops (Belalcazar et al. 2017; Sanchez et al. 2014). The XRF and NIRS based measurement methods are substantially less expensive than ICP or HPLC based methods.
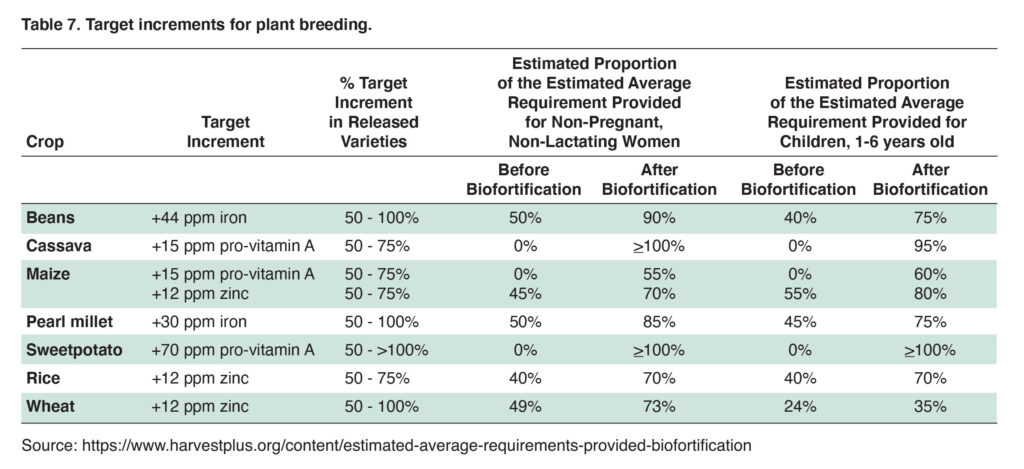
Conventional Plant Breeding
Figure 2 displays a conceptual framework for breeding biofortified germplasm and outlines the key activities in developing biofortified germplasm and reflects the impact pathway. The left column contains activities outside of crop development to ensure nutritional impact and farmer and consumer acceptance. The right columns reflect sequentially arranged stages and milestones in crop development, and are superimposed upon a decision-tree that allows monitoring progress and making strategic decisions if goals and targets cannot be achieved.
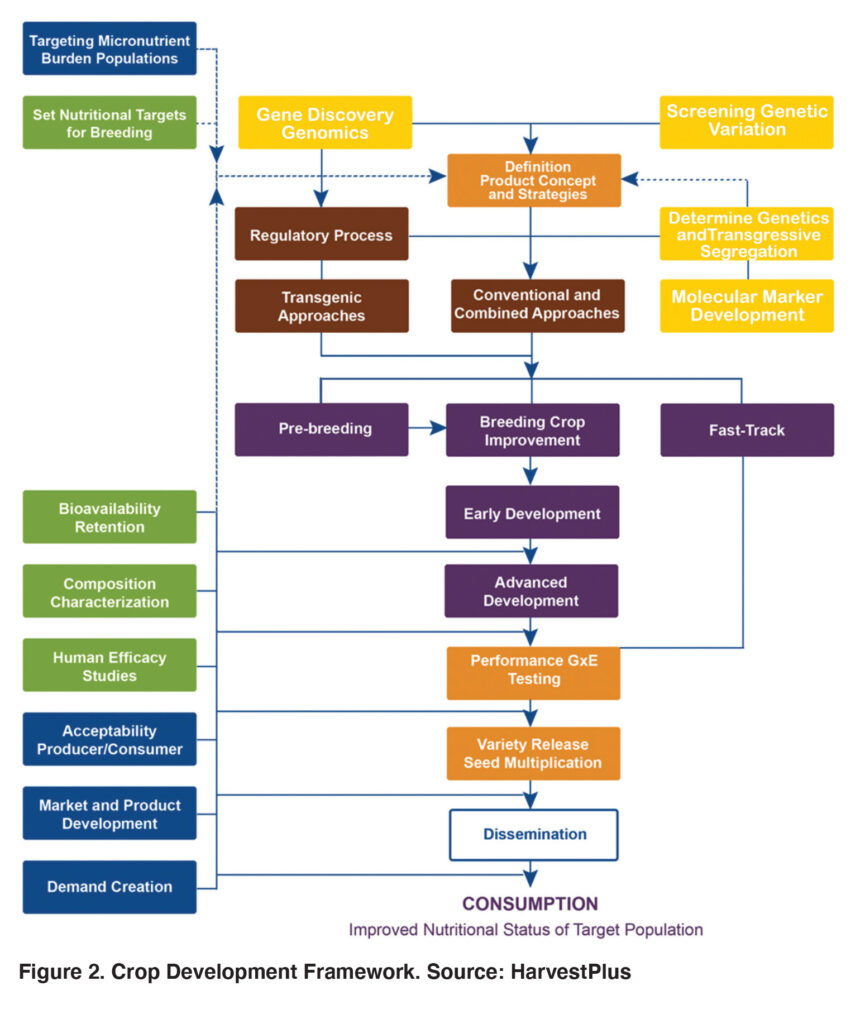
Crop improvement activities for biofortification focused initially on exploring the available genetic diversity for iron, zinc, and provitamin A carotenoids (yellow boxes) in the germplasm in ongoing breeding programs, and assessing diversity in the largely unimproved germplasm in core collections in gene banks, including wild relative species and unimproved stocks such as landraces. In parallel, in field evaluation or during subsequent screening, agronomic and end-use features are characterized, as varieties must provide good crop yields as well as marketing options to farmers. Objectives when exploring the available genetic diversity are to (1) identify micronutrient dense parental genotypes to be used in crosses, pre-breeding/parent building, development of molecular-markers associated with higher trait levels, genetic and crop physiological studies, and (2) identify existing varieties, pre-varieties in the breeding and release pipeline, or germplasm in final product development stage for “fast-tracking.” Identifying already developed varieties or hybrids and commercializing these genotypes that combine the target micronutrient density with the required agronomic and end-use traits enables “fast tracking” in which they are delivered immediately. If suitable variation for micronutrients is present only in unadapted sources in the strategic gene pool, pre-breeding is necessary prior to using the trait in final product development, as the trait needs to be combined with commercially used genetic backgrounds.
The difficulty in using unadapted sources in breeding may involve large genetic distances, the need for elimination of other unfavorable traits that initially come along with selection for the target trait, which adds to product development time and costs. If adequate variation is present in the adapted gene pool, the trait donors can be used directly to develop competitive varieties (purple boxes). Some breeding programs simultaneously conduct pre-breeding and product enhancement activities to develop germplasm combining high levels of one or more micronutrients. Once micronutrient density is available in adapted, high yielding background for target agro-ecologies and production conditions, the need for pre-breeding efforts decreases.
The next breeding steps involve: developing and testing micronutrient-dense germplasm, continuing to conduct genetic studies, further developing and using molecular markers for micronutrients, identifying loci for bioavailability and developing associated markers, genomic selection, and speed breeding with rapid generation advance to facilitate breeding progress (Gebremeskel et al. 2018; Guo et al. 2020; Mageto et al. 2020a, b; Menkir et al. 2018; Owens et al. 2014, 2019; Prasanna et al. 2020). In general, yield, agronomic and end-use characteristics are first considered in selection, as these traits trigger adoption by farmers and cannot be compromised. Breeding efforts for cross-pollinated crops focus on developing hybrids, but also can involve synthetics and open-pollinated varieties on a smaller scale during a transitional period, until formal and informal hybrid seed systems are established, become reliable, and hybrid seed costs are low enough to be accessible to small holder farmers. GxE—the influence of the long term climatic conditions and the more seasonal environmental factors on micronutrient expression—is then assessed at experiment stations and in farmers’ fields in the target countries (orange boxes), along with agronomic experiments to develop crop management recommendations for maximizing trait expression and yield. These experiments focus on sustainable agronomic practices such a minimum tillage, direct seeding, residue retention with reduced water use to enhance soil fertility and reduce the environmental footprint. The most promising varieties from multi-location testing over multiple seasons by national research partners, are then submitted to national government agencies for testing for agronomic performance and release, a process which typically takes two years, sometimes more. A key element in on-farm testing is Participatory Variety Selection (PVS). Farmers test the agronomic and end-use attributes of candidate varieties in PVS under their relevant situation and are genuine partners in breeding and guiding variety release recommendations.
International Nurseries/Global Testing
HarvestPlus has used two strategies to shorten time to market for biofortified crops: (1) identifying already adapted varieties with significant micronutrient content for immediate release and/or dissemination as fast track varieties, while varieties with target micronutrient content are still under development, and, (2) deploying multi-location Regional Trials with elite materials including released varieties across a wide range of countries and sites each growing season (Andersson et al. 2017). These regional or transcontinental nurseries serve in germplasm dissemination as well as a testing tool. Agronomic and micronutrient data from multiple sites per country allows high precision identification of fast-track candidates or inbred lines for breeding, it generates data on yield and micronutrient stability, and permits identifying the adaptive pattern of the germplasm to the different agro-ecological zones. By including end-use requirements, the germplasm can be grouped and targeted when tested in new countries. Further, by substituting assessment with temporal environmental variation with spatial environmental variation in large scale regional GxE testing, testing steps can be eliminated and time-to-market shortened by 1–2 years. Release in other countries is further accelerated by regional agreements, which harmonize seed regulations of member countries, and allow faster release if a variety has been released in similar agro-ecologies in one of the regional countries.
Strategic Priorities
The key longer term priority is mainstreaming biofortification. Mainstreaming refers to incorporating micronutrient density as a core trait in essentially all breeding programs developing varieties. Consequently all offspring, all future varieties will be biofortified and the breeding effort required will be reduced to maintenance breeding. Recent progress in developing molecular markers associated with higher levels of micronutrients will help facilitate mainstreaming (Babu et al. 2013; Swamy et al. 2016).
Medium term objectives aim at improving bioavailability of iron and zinc, for example by decreasing the anti-nutrient phytate, or increasing phytase, the enzyme that degrades phytate. For Vitamin A crops, breeding pursues increasing the retention and stability of provitamin A carotenoids.
Strategic priorities in breeding shorter term center on strengthening the pipeline of biofortified varieties by developing next waves of competitive, climate smart crops with target nutrient levels and broader adaptation across agro-ecological zones, production conditions and end-uses. Breeders can develop biofortified crops by directly breeding for increased micronutrient concentration, and/or higher bioavailability.
Releases of Biofortified Crops
Cumulatively, more than 400 biofortified varieties of 12 crops have been released in more than 40 countries, which includes all varieties facilitated by HarvestPlus plus orange sweetpotato varieties developed independently by CIP. Candidate biofortified varieties across 12 crops are being evaluated for release in an additional 20 countries.